Empirical approaches to bioreactor design fail to consider how the cumulative effects of stress and recovery as cells move around a stirred reactor affect cell productivity. TTP has developed CFD modelling techniques to predict how bioreactor design can affect cell culture productivity.
Context
A well-known challenge in the design, validation and reliable operation of bioreactor systems is scaling-up from laboratory ‘litre scale’ reactors to those operating at an industrial scale.
Solution
TTP’s CFD modelling techniques take bioreactor development beyond trial and error by elucidating how exposure to mechanical stress and nutrient micro-environments in different reactor designs impinges on cell productivity and vitality.
Result
TTP’s methods provide a route to rapid development and efficient exploitation of valuable cell cultures, by showing how standard elements in traditional bioreactor design affect the productivity responses of cells.
Cells in bioreactors are sensitive to a wide range of influences which can, either singly or in combination, reduce cell culture productivity.
Influences that may disturb cells include physical damage due to agitation, inadequate nutrient supply, unfavourable temperature or waste accumulation, population dynamics, diseases and foreign invasions.
In addition, biological studies show that cell productivity can be affected by prolonged low-level shear, but cells are also capable of recovering from, and may even be stimulated by, certain levels of mechanical stress.
As reactor size increases, so does the power of the agitators however. This raises the risk of mechanical stress and cell damage, thus forfeiting potential productivity gain from moving to a larger system.
Crucially, traditional empirical approaches to bioreactor design cannot anticipate how the cumulative effects of stress and recovery as cells move around a reactor might affect biological productivity.
Our CFD modelling techniques achieve this by first simulating the flows and shear histories experienced by hypothetical tracer cells inside different bioreactor designs and then using these shear history traces to drive a biological damage-and-recovery model to estimate cell performance in different reactor designs.
Take, for instance, a cylindrical reactor design with two impellors where the main variable is the angle of the impellor blades, i.e. a pair of radial Rushton impellors or a pair of axial impellors in the same tank.
The paths followed by cells in these reactors are revealed by tracer particles, whose exposure to shear and other variables can be mapped out and analysed.
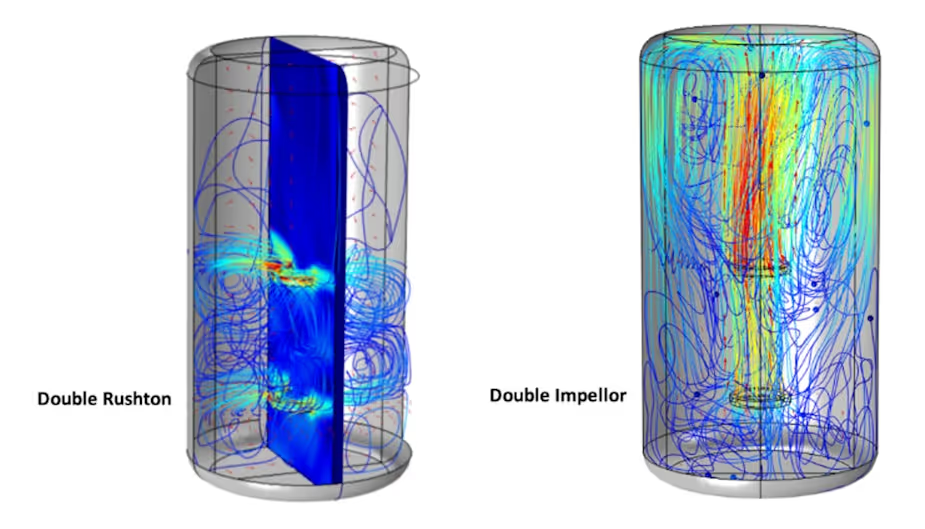
Our models allow us to quantify differences between these systems in terms of peak shear values, the frequency of their occurrences and the durations of shear stress experienced by tracer cells. This reveals markedly different shear histories in the two bioreactors.
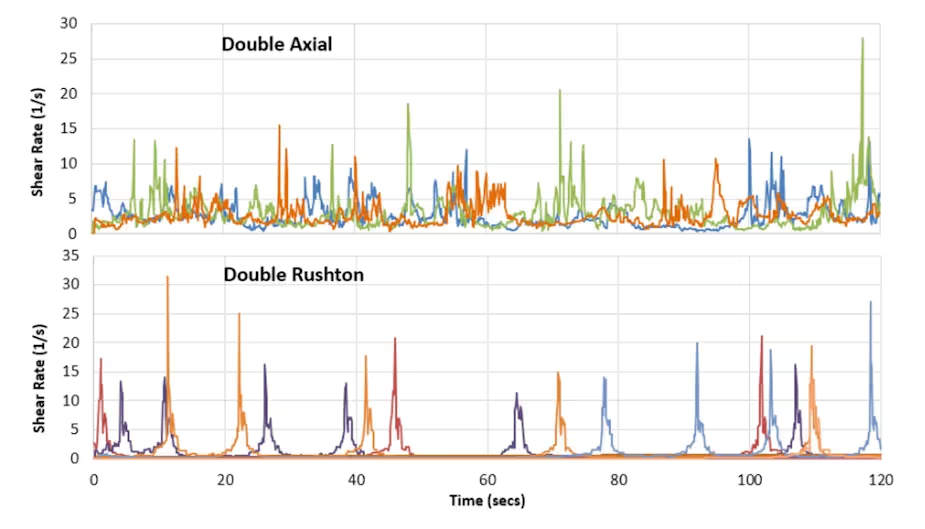
Figure 3 shows the results of combining this data with a shear damage-recovery model. In the example, cells in the radial Rushton impeller-driven reactor recover from periodic shear stress, thanks to intervening periods in environments of relative calm, while cells in the double axial impeller reactor suffer escalating shear stress.
We are thus in a position to make specific estimates of cell viability and productivity in different bioreactor designs.

We have also used this approach to map out and analyse the fluctuating nutrient micro-environments as well as temperature and gas concentrations experienced by tracer cells, thus inferring single-cell stress and other micro-response variables from macroscopic reactor design elements.
These results can be fed back into bioreactor design in order to achieve environmental exposure profiles more appropriate to cell requirements. Our CFD models thus provide a route to rapid development, optimised scale-up and efficient exploitation of valuable cell cultures.