Is ultrasound the key to powering the next generation of implants?
By Alon Greenenko
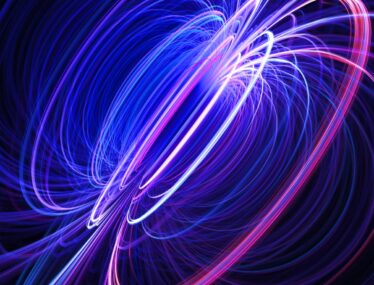
Ultrasound can be used to charge and power even the smallest implants that are out of reach for inductive power transmission methods. Some implant developers have adopted this approach already, but challenges remain. How will we be able to take full advantage of ultrasound to power future implants?
Active implanted medical devices like pacemakers and neurostimulators are packed with functionality and grow smaller with each generation. But as the size of these devices becomes comparable to the size of a pill, and implantation sites move from superficial layers to inner organs deep within the body, the conventional ways of powering implants reach their fundamental limits.
In this blog, we discuss the main ways of delivering energy to active implants, namely:
- Batteries
- Electromagnetic powering
- Ultrasonic powering
Batteries
Long lifetime batteries quickly became the standard way of delivering power to implanted systems such as cardiac pacemakers [1, 2]. The key attraction of primary cell batteries is the low user burden, as they can last many years without replacement or recharging, particularly when the therapeutic power consumption is low.
For implants that require more power, rechargeable secondary cell batteries can be used. These need to be topped up regularly using an external power source, but when the charging intervals reach days or weeks the added user burden remains relatively low. This approach is commonly used in deep brain stimulation systems to treat Parkinson’s disease, spinal cord stimulators for pain treatments and other non-life-critical devices.
Unfortunately, despite advances in technology, batteries remain a relatively large component of active implants, and an obstacle to implant miniaturisation. Whilst in the short term we may see a small reduction in the size of rechargeable batteries, in order to miniaturise implants further we need to look beyond batteries to inductive power links and ultrasonic approaches.
Electromagnetic powering
The most common method of wireless power transmission is through magnetic induction, as seen in consumer products such as electric toothbrushes and mobile phones. The same technology can be applied to recharge batteries in implanted medical devices, or even to provide immediate power to implants from a power source outside the body.
Induction is easy to use due to its contactless nature and the fact that it can transmit power through air and tissue without having to make a direct physical contact with the skin. It is also relatively safe: if the receiving coil on the implant is sufficiently large, it is possible to design a system that delivers the required power without causing any damage to the body. This is helped by the fact that inductive systems operate in frequency bands where the energy absorption in tissue is low.
However, as implants become smaller and implantation depths increase, inductive powering starts to struggle to deliver enough power because the fundamental principles behind inductive power transfer require the receiver coil to be relatively large, close to the transmitter, and well aligned to the transmitter.
Radio Frequency (RF) approaches, which use higher ISM frequency bands for electromagnetic energy transfer, can overcome the coil size limitation. Studies have shown that RF-based systems can deliver similar power levels to inductive powering even when the coil area of the receiver is a hundred times smaller [3-6].
Yet even RF approaches are limited in the total power that they can deliver to an implant. This is due to a higher tissue absorption rate that rapidly increases as a function of frequency, limiting the power that can be safely transmitted without causing harm to the tissue.
Owing to these fundamental limits, some implant developers are leaving electromagnetic power links behind and opting instead for ultrasonic powering.
Ultrasonic powering
Ultrasound has a long history in medical applications, ranging from medical imaging to surgical ablation. The same technology can also be used to wirelessly transmit power to small implants. One example is the WiSE CRT system, where a cardiac implant the size of a grain of rice (just 2×10 mm) is powered by an ultrasound transmitter placed just under the skin [7].
Ultrasonic powering has the advantage of significantly lower tissue absorption compared to RF approaches, meaning it can safely deliver more power without risking tissue damage. This enables ultrasound to reach much deeper implants compared to RF. In addition, ultrasound has much shorter wavelengths in tissue than RF, which means that with appropriate beam forming techniques it can be focused onto sub-millimetre receiver elements to power pill-sized implants.
Despite these significant advantages, there are two obstacles to ultrasonically charging implants from outside the body.
First, coupling ultrasound into the body presents a potential usability challenge. Any air trapped between the skin and the ultrasound transducer will have a detrimental effect on the efficiency of power transfer. This is the reason why ultrasound imaging operators apply copious amounts of gel and press the probe very firmly against the skin. Whilst this is acceptable in a clinical situation it is clearly impractical for home use. Therefore a crucial element for future ultrasonic charging systems is a user-friendly coupling interface that avoids any need for gels. Flexible polymers are good candidates for solving this challenge.
A second challenge is achieving the required alignment between the ultrasound beam and the receiver elements within the implant. During ultrasound imaging this task is performed by hand to find features of interest, but for at-home powering this process needs to be fully automated. This can be achieved using ultrasonic beam forming techniques and sophisticated algorithms to steer and focus the ultrasound energy on the target implant area.
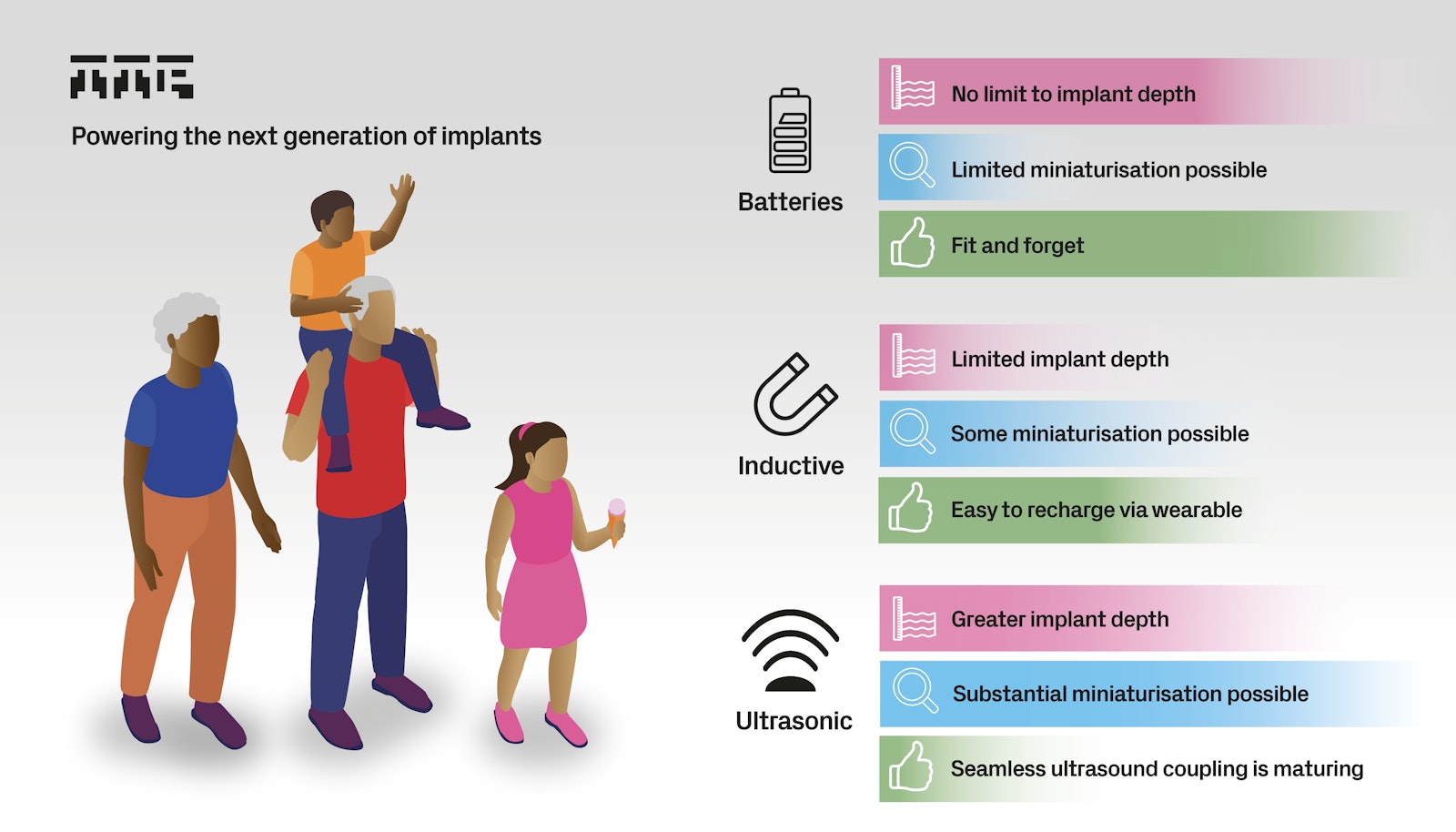
Outlook
The challenges of seamless through-body coupling, alignment, compensation for body movement, and efficient transmission of ultrasound through both tissue and implant enclosures mean that ultrasound powering systems need to be tailored for each specific use case.
But solutions to these challenges will enable a new class of long-term implantable devices whose smaller size can deliver innovative sensing and therapy platforms to organs that are not currently accessible.
TTP designs and develops next-generation medical implants using a holistic multi-disciplinary approach to identify custom solutions for each specific case. We run development programs from specification of top-level requirements through to setting up supply chains and transfer to manufacturing. We aim to achieve tight integration of powering with other implant sub-systems and, ultimately, optimum implant design.
How we help our clients | Case study: Developing next-generation neuromodulation therapy products | Healthcare Case Studies | Healthcare Blogs