How small can we go? Designing the next generation of implantable neurostimulators
By Seoirse Murray
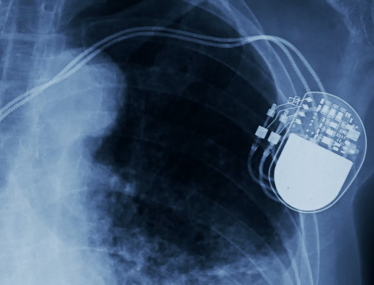
The trend towards greater miniaturisation offers opportunities for innovation within the implantable pulse generator (IPG) design space. It has put renewed focus on the design of the electronics and mechanical packaging. Delivering a market-leading IPG with the smallest volume requires a multi-disciplinary approach that creatively considers functionality and manufacturability across multiple domains.
In this blog, we discuss, why and how to make smaller implantable neurostimulator? can we do without batteries, and what would this mean for patients? And what are the technical challenges of smaller device design?
What is a neurostimulator?
Neurostimulators are implanted medical devices that treat long-term conditions, such as chronic pain or Parkinson’s disease, by delivering electrical current to nerves in the spinal cord, brain or peripheral nervous system. Neuromodulation offers an alternative to traditional drug treatment, with an entirely different mechanism of action which can avoid some of the side effects common to pharmaceuticals such as opioid addiction. As such, interest in neurostimulation therapy is growing, and devices are increasingly being applied to other diseases including epilepsy, OCD and auto-immune disorders.
Neurostimulation systems consist of an IPG to generate the electrical current, a set of leads to transmit this from the IPG to the target site, and an array of electrodes which directly interface with the nerve bundle and deliver the current into the nerves. There can also be a handheld programmer or app to communicate with the IPG to control its settings.
Why go smaller?
As with any implant, there is a desire to minimise the IPG’s size to reduce the invasiveness of the surgery and minimise the long-term impact on the patient. Having a large, hard object in the body can be uncomfortable in daily life. Depending on the patient’s body type, a large IPG can even be visible under the skin. Smaller implants are less likely to be accidentally displaced or rotated in the body while also enabling a greater choice of implant sites. In general, a reduced size of implant carries fewer risks and drawbacks, thereby opening new treatment options to patients with less severe symptoms. Miniaturisation of neurostimulators is particularly challenging as they contain a lot of functionality, and can be proportionally larger than many other implantable devices.
How can we go smaller?
The battery that powers a neurostimulator is often the largest single component and ends up dominating the overall volume of the IPG. Other than using more energy dense battery types, the only way to reduce this volume is by reducing the power requirements. Careful design of the electronics can reduce the idle power draw of the electronics, and stimulation waveforms can be optimised to reduce the active draw. A 10 µA reduction in current draw over a 10-year lifetime equates to a significant volume of battery!
To reduce this volume further, some newer IPGs use rechargeable batteries which can be one quarter of the size of a non-rechargeable equivalent. They are recharged through an inductive link, a pair of coils over which electromagnetic energy is transferred wirelessly. This is the same mechanism that is used for wirelessly recharging your electronic toothbrush or mobile phone.
To take this approach one step further, it is possible to completely remove the battery from the implantable part of the device. As with a cochlear implant, which is used to provide a sense of sound to people who are severely hard-of-hearing, the IPG could then be powered from an external battery over an inductive charging link. In making this change the potential size of the IPG can be dramatically reduced. However, ensuring a reliable and efficient wireless link to the device becomes very important to ensure consistent therapy.
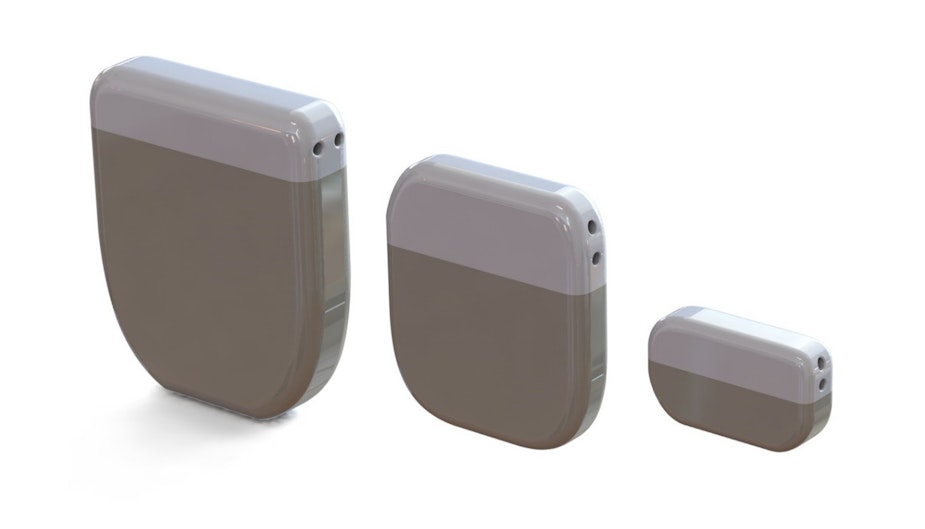
Figure 1: From left to right, a primary cell, rechargeable, and externally powered IPG showing relative sizes
What will wireless charging mean for therapies?
There are many benefits to having an IPG which is as small as possible, however these benefits must be carefully balanced against the requirements of a given therapy. Critical-to-life devices should not be reliant on an external charging device or power source. In these situations, the safety of having a primary cell device of a known lifespan greatly outweighs the increased inconvenience and surgical risk. For instance, a pacemaker should carry a primary cell as the consequences of forgetting to charge it are so high!
For therapies which are not critical to life, but which require near constant application, a rechargeable device often makes sense. This allows a substantial reduction in the size of the implantable part of the system and often an increase in its lifetime. It does require the user to remember to charge the device regularly, but the cost of forgetting is not disastrous. This approach is often applied for pain therapies such as spinal cord stimulation.
There are applications for which an entirely externally powered device is the best choice. If the device is in an area of the body with limited space, this may be the only option. In the case of a cochlear implant an external microphone is required, so the marginal cost of also making the device battery external is small. This is compounded by the space requirements of the area around the skull. Could the same approach be applied to IPGs elsewhere in the body?
What is the impact on patients?
As with any medical device it is critical to consider the implications of design changes on the way in which a user will interact with the device. How will users respond to wearing a visible external device to provide power, and how often will they be willing to do this?
To achieve a strong communications link for charging or stimulation it is important to maintain a good alignment between the IPG and the wearable. Making this wearable light and comfortable across a wide range of potential body shapes and sizes – while simultaneously holding the internal and external coils in close proximity as the user moves – is not a trivial problem. It can be further compounded by the likelihood of patients having other conditions like poor mobility or dementia which may hinder use of the device.
This is where a consideration of how the neuromodulation therapy is actually used and how the patient would prefer to interact with it, is pivotal for good product uptake and a positive patient experience.
What are the technical challenges of ever smaller device design?
When the use case is best suited to a rechargeable or externally powered device this has some implications on the design of other sub-systems. With the battery volume reduced or even removed altogether, greater thought needs to be put into the design of the other components and how they integrate. If the battery no longer constrains the size of the IPG, other parts of the system design become more critical. The electronics, mechanics, and comms must be considered holistically to minimise the overall device dimensions through reduced component size and minimal wasted volume.
Electronics design needs to adapt to an intermittent power regime where more processes require frequent, robust and cyber-secure communications between the wearable and implantable parts of the system. Inefficiencies in power consumption in the IPG are amplified over the wireless power transfer link making current draw in the IPG, as well as efficient and matched inductive coil circuits, the dominant factors in the charge-lifetime of the system.
An application specific integrated circuit (ASIC) can be used to achieve the lowest possible electronics footprint. This can be nearly fully self-contained, often only needing a few external capacitors to implement complex mixed signal designs in a power and volume efficient way. This approach is not without the setbacks of high NRE and long development timelines, but alternative designs using system-on-package PCB technology with embedded discrete components can also achieve considerable space savings. In these situations, laying components out so that they tesselate efficiently in 3D with other IPG components while fitting within the process guidelines can greatly aid miniaturisation of the whole system.
With the electronics shrinking in size, the mechanical design must adapt to suit. What previously may have been a straightforward packaging exercise has renewed emphasis because of the reduced scale; a simple bolt may now represent a reasonable fraction of a sub-system’s volume. To minimise these volumes, the mechanical packaging must find ways to “catch up” with the electronics in scale. Some avenues include new manufacturing methods that enable smaller components, and novel ways of interfacing with the leads to shrink overall volume. For example, can we combine the functions of sealing and retaining the lead in the IPG into a single component? This sort of creative thinking will be pivotal in minimising the overall volume of IPGs in the future.
Why TTP?
TTP have the multi-disciplinary expertise to develop the next generation of IPGs, designed for low power and small size, bringing together a bottom-up understanding of the fundamental circuits with a holistic approach to the product requirements and packaging design. This multi-faceted, multi-disciplinary approach enables the tight integration of optimised sub-systems and, ultimately, the optimum IPG design for a given application and use case.